Meet our PhD candidates within the EU Marie Sklodowska-Curie Program (MSCA) COFUND trainLSE project
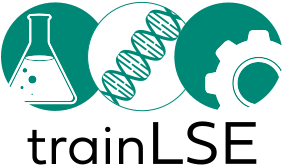
The program “Interdisciplinary and intersectoral doctoral training programme at Technische Universität Darmstadt in Life Science Engineering” – trainLSE – aims to establish interdisciplinary research and training of excellent international young researchers at the interface between engineering and life sciences. trainLSE is integrated into the existing PhD program of the Graduate School Life Science Engineering (GS LSE) at TU Darmstadt. PhD candidates are members of the LSE community and benefit from the structured doctoral training.
The MSCA-COFUND doctoral program not only provides research training in the fields of biology, chemistry, materials science, electrical engineering, physics, and mechanical engineering, but also prepares you for your future career in academia and industry and offers the opportunity of research internships in renowned partner research institutions, universities or companies in different countries.
Our final 10 candidates were selected in April 2024 and started their exciting journey at TU Darmstadt:
Name | Lab | Department |
Tarik Benali | Self-Organizing Systems | Prof. Koeppl – ETIT |
Georgie Solyus | Self-Organizing Systems | Prof. Koeppl – ETIT |
Farah AlHamam | Chromosome organisation & Centromere identity |
Prof. Heun – Biology |
Gizem Cantörü | Biohybrid Systems for Cellular Engineering | Dr. Belluati – Chemistry |
Ezgi Sentürk | Integrated Micro- and Nanosystems | Prof. Burg – ETIT |
Lakshmy T Ajithan | Integrated Micro- and Nanosystems | Prof. Burg – ETIT |
Livia Campos | Biophotonics – Biomedical Engineering | Prof. Frosch – ETIT |
Salomé Luis | BioMedical Printing Technology | Prof. Blaeser – Mechanical Engineering |
Esraa Elmorsy | Nanostructures fabricated by ion-track technology | Prof. Toimil-Molares – Material Science |
Mohammadjavad Jazini | Control and Cyber-Physical Systems | Prof. Findeisen – ETIT |
The journey to Darmstadt
Our journey began in February 2024 when we met selected candidates for virtual interviews. The top candidates were then invited to Darmstadt for an on-site recruitment event, which included a joint dinner and a campus tour of our university.
In January 2025, the coordinator held a welcome session for the trainLSE Fellows. This included a comprehensive introduction to the trainLSE training programme, as well as an opportunity to get to know each other better and expand their professional networks.
Looking ahead, we have many exciting events and training sessions planned for 2025. Stay curious—we'll keep you updated.
November 2024: Nature Masterclass on Scientific Writing
The LSE hosted an inspiring Nature Masterclass on Scientific Writing in Darmstadt, offering an exceptional opportunity for its members to sharpen their academic writing skills. This exclusive workshop, open to all LSE members including participants in the trainLSE program, was led by two experienced editors from Nature.
During the workshop, participants gained valuable insights into the (often challenging) peer-review process and learned key strategies for creating compelling research papers. The editors shared practical tips, behind-the-scenes perspectives, and concrete advice on how to make manuscripts stand out.
A networking dinner rounded off the event, providing the perfect setting for informal conversations where students could engage directly with the editors, ask questions and exchange ideas in a relaxed and friendly atmosphere.
February 2025: Visit to GSI Helmholtz Centre for Heavy Ion Research in Darmstadt
Our regular LSE Seminars provide a fantastic opportunity for students to engage with the community and organize exclusive excursions. Recently, a PhD student from the Graduate School arranged a guided visit to the GSI Helmholtz Centre for Heavy Ion Research in Darmstadt. This included a tour of the FAIR international accelerator facility, one of the world's largest research projects. It was an incredible chance to explore cutting-edge accelerator technology and its role in advancing scientific discovery.
April 2025: First Industry talk by PAiCON
During our annual retreat in the beautiful Kleinwalsertal, Austria, we had the great pleasure to get to know more about an inspiring company from Heidelberg called PAiCON.
Dr. John Wong was invited to join us and gave an instriduction about the innovative work of PAiCON – a young start-up using AI-driven data anylsis to support early and accurate cancer diagnostics.
The industry session are an important part of the trainLSE programme. They give our fellows an insight into research in industry and demonstrate possible career prospects after completing their PhD.